Very many important bio-molecules— from the smallest building blocks like amino acids, through larger proteins, to the famous DNA double helix— are chiral, meaning that like our hands they can possess a distinct handedness. Most instrumental techniques cannot distinguish between the left- and right-handed mirror image structural forms of a chiral molecule, but because the biochemistry of life is inherently chiral, living organisms can readily do so.
Examples include our ability to distinguish between a chiral pair by perceiving their different odours. Insects similarly distinguish the chiral forms of pheromones. And the pharmaceutical action of many drugs critically depends on their chiral form. Underpinning understanding of this biological sensitivity to handedness is the “lock and key” principle, which argues that an enzyme or reagent interacting with a substrate must have the right shape to fit into a specific interaction site. In the case of a chiral substrate this requires a matching chiral form — rather like finding that only a right hand can be comfortably fitted into a right-handed glove.
A long-standing collaboration between scientists from the University of Nottingham (UK) and the DESIRS group at SOLEIL has been responsible for introducing and developing a technique, Photoelectron Circular Dichroism (PECD) that exploits the ability to produce circularly polarized (left and right handed) synchrotron radiation to probe sensitively molecular handedness. Specifically, PECD observes the asymmetric motion of electrons through a chiral molecule upon photoionization. In a recent study published in Angewandte Chemie, the team examined a number of small diols (double alcohols). The smallest considered, 1,3 propanediol, consists of a three membered carbon chain with an alcohol (OH) group attached at either end. In one sense this is a fairly symmetric arrangement (so a priori non-chiral), but the OH groups prefer to align themselves head-to-tail creating the possibility for a mirror image pair (see Figure). The outermost (HOMO) orbital reflects this chiral mirroring! However, rotation of the OH groups is facile, so that these forms rapidly interconvert. Propanediol’s chirality is therefore only transient and the distinct forms cannot be isolated. Of course, interactions with a chiral substrate could stabilise or lock the OH rotation, and in such circumstances propanediol could adopt whichever handedness was preferred by the interaction.
Adding a methyl group to one end next produces 1,3 butanediol, and this slows down the rapid OH rotation. It also breaks the strict mirror image symmetry, and yet the head-tail arrangements, or conformation, of the OHs and the shape of the HOMO electron orbital are seemingly unaffected. Locally, the possibility for two chiral forms is retained (see Figure). But the additional methyl group in butanediol has another consequence; it creates an asymmetric substitution at what was the terminal C(3) atom, which thus itself becomes a permanent chiral centre. Considering the bond arrangements (configuration) along the carbon chain, the 1,3 butanediol structures shown in the figure here are both the same (right handed) form of this new permanent chiral pairing, which can now be isolated for commercial samples.
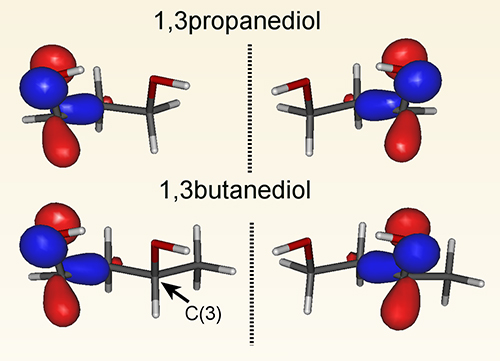
Figure: Orientation of the OH groups in propanediol creates a mirror image symmetry, and the HOMO orbital shape shown also reflects this chirality. This handedness persists as a pseudo-mirror imaging when a methyl is substituted to create 1,3 butanediol. However, this asymmetric substitution breaks the strict mirror imaging in the figure and also creates a new, permanent chiral centre at the C(3) atom. An alternative methyl substitution at the C(3) site, made from the front in this figure, would leave the asymmetrically substituted carbon having a permanent configuration of the opposite handedness.
The 1,3 butanediol molecule hence possesses two types of chirality; a permanent configurational chirality (that can only be switched by breaking and reforming bonds), and a conformational chirality (that can be exchanged by rotating bonds). Which is responsible for determining the left or right handedness sensed in a molecular reaction? Using PECD, in a combined experimental and numerical modelling study, the team found that conformation of the OH groups still provides a dominating contribution to observed chiral asymmetry when the HOMO electron is excited and ionized, i.e. the conformational chirality is not quenched by the more permanent configurational chirality of the carbon framework. It is just this response of the outer electron that is typically sensed by a reaction partner. This finding is important because its suggests how changes in the conformational chirality, readily induced by relatively weak interactions in the process of reagent-substrate docking, might sometimes prove more significant than, and so override, the more permanent configurational molecular chirality.