Nuclear energy is the largest source of electricity production in France. In order to reprocess the spent nuclear fuel, fission products and minor actinides are concentrated and mixed with melted borosilicate glass. Scientists from the “Laboratoire des Matériaux et Procédés Actifs » group (CEA, Marcoule) performed X ray spectroscopy absorption experiments on the MARS beamline, in order to study the effects of α-radiation on the molecular structure of borosilicate glasses.
France has decided to reprocess the spent fuel originating from commercial nuclear power plants prior to the long-term storage of the fission products (FP) and minor actinides (MA) extracted from the irradiated fuel. FPs and MAs, representing less than 5% of the weight of spent nuclear fuel, are concentrated into solution and then mixed simultaneously with melted borosilicate glass (figure 1). As a result, FPs and MAs are not only trapped inside the glass but also form bonds which become part of the glass network. The overall waste management industrial process was developed by AREVA and implemented at “La Hague” facility. It ensures the reliability and durability properties of the stored nuclear material over several hundred thousand years.
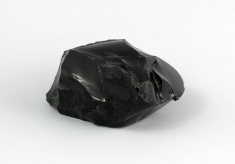
Figure 1: Industrial nuclear waste glass composed of more than thirty oxides.
Simulation in the lab
On the laboratory-scale, to better understand physical phenomena, it is possible to study the behaviour of simplified nuclear glass under self-irradiation effects, for example if the glass is doped with an actinide element, such as curium. Curium-244 has a half-life of 18 years and mainly decays to plutonium by emitting an alpha-particle with an average energy of 5.8 MeV.
To better understand self-irradiation effects from the structural point of view, we performed XAS experiments on the MARS nuclear beamline at SOLEIL to analyse three different samples:
- an inactive six-oxide borosilicate glass (ISG) considered as a reference
- a Cm-doped borosilicate glass (D-ISGC, with the same composition, see table 1) melted eight years ago, which has undergone α-decay damage until reaching a stabilised structural state
- an annealed sample (A-ISGC), taken from the previous batch, in which the thermal cycle has erased all structural damage.
Both the latter samples had a cumulative radioactivity close to 2.104 times the radioactivity at the exemption threshold (which is the actual authorised limit on the MARS beamline).
Table 1: Compositions of glasses. t0 reflects the initial time corresponding to ISG glass melting. D-ISGC refers to the damaged glass.
Oxide wt% | SiO2 | B2O3 | CaO | Na2O | Al2O3 | ZrO2 | Cm2O3 | PuO2 |
ISG | 56,18 | 17,33 | 4,98 | 12,17 | 6,06 | 3,28 | 0 | 0 |
ISGC (t0) | 55,32 | 17,07 | 5,01 | 12,0 | 6,03 | 3,26 | 1,02 | 0,29 |
D-ISGC (t0+8 years) | 55,32 | 17,07 | 5,01 | 12,0 | 6,03 | 3,26 | 0,85 | 0,46 |
Disorder increases around Zr
We chose to characterise the local environment of curium (Cm), plutonium (Pu) and zirconium (Zr) species present in the glasses using the XANES technique. In particular, it is worth noting that it was the first time that Cm element was investigated by XAS on the MARS beamline. Fluorescence mode was used to avoid the proximity of absorption edges for Pu and Zr species, at the L3 and K edge respectively. The L3 edge was also used for Cm species. The XANES spectral region can give us structural information, but in practice, due to interpretation difficulties, XANES features mainly tell us about the local symmetry around the absorber atom and its electronic structure.
From the fluorescence XANES spectra, we concluded that no change occurred in the Cm local environment. Furthermore, an interesting result relating to the spectra at the Zr K-edge (figure 2) was obtained. In this respect, we tried to assess qualitatively the proportions of the sites of symmetry for the different glasses ISG, D-ISGC and A-ISGC, by combining elpidite and m-ZrO2 XANES spectra, seen as crystalline structures where Zr is found respectively in 6-fold and 7-fold coordination sites. The major component in ISG was around 85% elpidite with a low m-ZrO2 component of 15%. The elpidite component in D-ISGC decreased to 58% and for A-ISGC it was close to 10%. Therefore, a decrease in the D-ISGC octahedral component (6-fold symmetry site) reflects an increase in disorder related to α-self irradiation effects around Zr species. Moreover, from 11B NMR experiments on the same glasses, we know that BO4 structural units decrease within a few percent, enabling more light alkali ions to move over the silicate network. Therefore, this physical phenomenon allows more neutralisation of large negatively charged structural entities built upon Zr species.
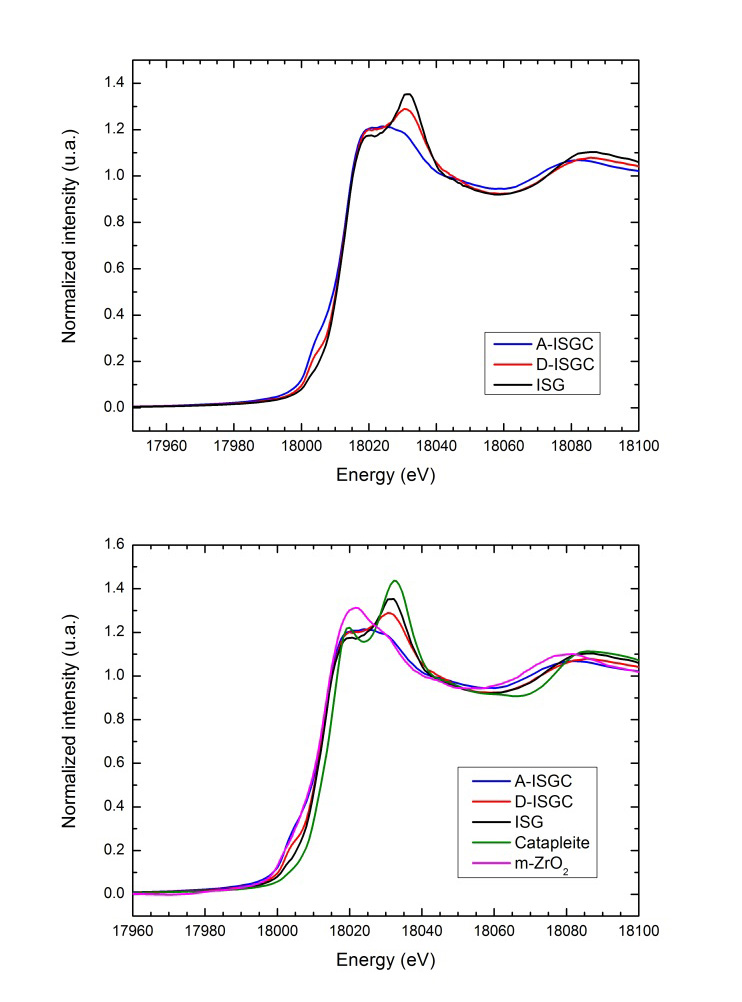
Figure 2: (top) Fluorescence XANES spectra at Zr K-edge for D-ISGC (red curve), A-ISGC (blue curve) and for the inactive glass ISG (black curve); (bottom) Comparison of XANES spectra at Zr K-edge for the D-ISGC (red curve), A-ISGC (blue curve), ISG (black curve) with crystalline references catapleite (green curve) and m-ZrO2 (magenta curve).
On the other hand, the annealing process failed to retrieve the initial ISG structure, as more disorder was introduced around Zr species (see figure 2). However, in contrast, 11B NMR informs us that the initial [4]B proportion was recovered in the annealed sample. Consequently this means that there should be a change in silicate units, as more alkali or alkali-earth ions are needed to act as charge-compensators for large Zr-based entities. More generally, it seems likely that the annealing process succeeds in recovering initial borate units but not silicate ones.
Further experiments are under consideration for the future that will combine Wide Angle X-ray Scattering (WAXS) and atomic-level simulations, to better describe local environment and medium range order around the different heavy species present in the nuclear glasses.