Iron-sulfur clusters are ubiquitous cofactors made of iron and inorganic sulfur. They are essential for the function of proteins involved in a great range of activities: electron transport in respiratory chain complexes, regulatory sensing, photosynthesis, response to oxidative stress, free iron ions supply, DNA repair… In most iron-sulfur proteins, the cluster(s) acts as electron-transfer group in mediating one-electron redox processes. Scientists from Florence University studied the recognition between 2 human key proteins involved in the assembly of the Fe-S clusters.
Several diseases linked to defects in the Fe-S cluster biogenesis
It is now evident that several rare and apparently dissimilar human diseases are attributable to defects in the basic process of Fe-S cluster biogenesis. Although these diseases (which include Friedreich’s ataxia, ISCU myopathy, a rare form of sideroblastic anemia, an encephalomyopathy caused by dysfunction of respiratory chain complex I and multiple mitochondrial dysfunctions syndrome) affect different tissues, a feature common to many of them is that mitochondrial iron overload develops as a secondary consequence of a defective Fe-S cluster biogenesis.
Therefore the understanding of the iron-sulfur cluster biogenesis is of key relevance. As the proteins involved in the biogenesis of Fe-S clusters are evolutionarily conserved from bacteria to humans many molecular details on the process of Fe-S cluster biogenesis come from studies of model organisms, including bacteria, fungi and plants.
Complex machineries are required for this biogenesis
Because inorganic sulfide and ferrous/ferric iron atoms are toxic in vivo, biogenesis of iron–sulfur cluster proteins is a highly regulated process that requires complex protein machineries. In the cytosolic iron–sulfur protein assembly machinery, two human key proteins NADPH-dependent diflavin oxidoreductase 1 (Ndor1) and anamorsin form a stable complex in vivo that was proposed to provide electrons for assembling cytosolic iron–sulfur cluster proteins. The Ndor1–anamorsin interaction was also suggested to be implicated in the regulation of cell survival/death mechanisms.
In the present work the scientists unraveled the molecular basis of recognition between the FMN-binding domain of Ndor1 and of the C-terminal region (linker and CIAPIN1 domain) of anamorsin containing the [2Fe- 2S] cluster in the oxidized state and of the electron transfer process. This is based on the combined NMR and X-ray (experiments carried ou on PROXIMA1) structural characterization of Ndor1 (Fig. 1) and anamorsin, on the investigation of the electron transfer process (Fig. 2), and on the identification of those protein regions involved in complex formation and those involved in electron transfer.
The scientists found that an unstructured region of anamorsin is essential for the formation of a specific and stable protein complex with Ndor1, whereas the C-terminal region of anamorsin, containing the [2Fe-2S] redox center, transiently interacts through complementary charged residues with the FMN-binding site region of Ndor1 to perform electron transfer.
The molecular model of the proposed electron transfer process provides significant information on the functional processes in which Ndor1-anamorsin interaction is implicated, i.e., the assembly of iron-sulfur clusters (1), diferric proteins (2) and the regulation of cell survival/death mechanisms (3, 4). Indeed, the disruption of the stable interaction between anamorsin and Ndor1 might provoke the interruption of the electron flow between the two proteins within the cell and, as a result of that, its essential function for cellular survival is abolished and consequently cell death mechanisms might be activated.
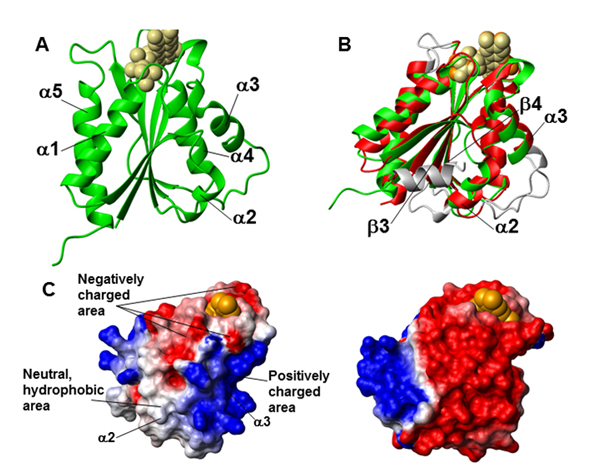
Fig. 1. Crystal structure of the FMN-binding domain of Ndor1. (A) Ribbon representation of the crystal structure of the FMN-binding domain of Ndor1. FMN is shown as beige spheres. (B) Superimposition of the crystal structures of the FMN-binding domains of Ndor1 (green, FMN in beige spheres) and of the human NADPH-cytochrome P450 reductase (PDB ID: 1B1C) (red, FMN in orange spheres). The regions with structural variations between the two proteins are shown in gray. (C) Molecular surface of the FMN-binding domain of Ndor1 (Left) and of the human NADPH-cytochrome P450 reductase (Right) colored according to their electrostatic potential. The views are equivalent in terms of the orientation of the protein backbone. The colors of the molecular surface indicate positive (blue), neutral (white), and negative (red) electrostatic potentials. The FMN molecule is shown as orange spheres.
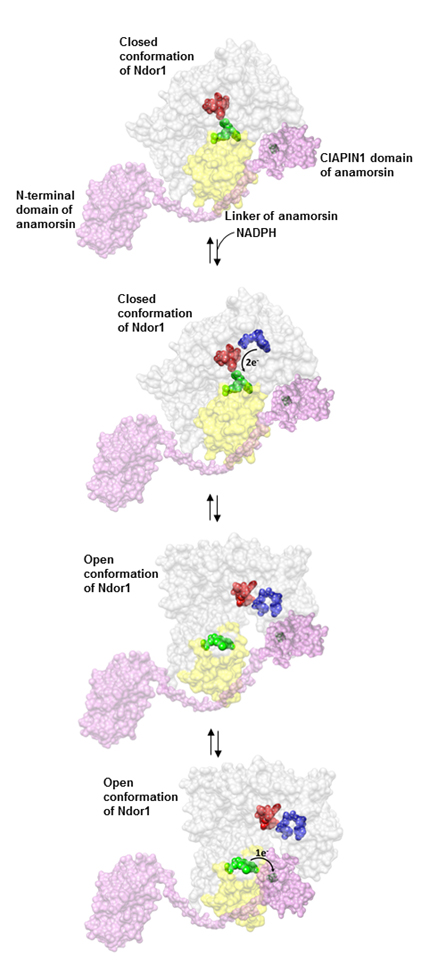
Fig. 2. Model of the electron transfer process between Ndor1 and anamorsin. Anamorsin (in pink) can be tightly bound to both closed and open confor- mational states of Ndor1 (in gray) due to the specific recognition between an unstructured region of anamorsin and a region of the FMN-binding domain that is solvent exposed in both open and closed conformations (in yellow). The N-terminal domain of anamorsin is not involved in the protein–protein recognition process. Upon NADPH (in blue) binding, electrons can efficiently be transferred in the closed conformation of Ndor1 to FAD (in red) and then to FMN (in green) to produce the hydroquinone state of FMN. Interflavin electron transfer in Ndor1 significantly populates the open conformation, which exposes to the solvent the FMN moiety and allows the formation of a transient interaction with the [2Fe-2S] cluster region of anamorsin located in the CIA- PIN1 domain. The latter interaction allows an efficient transfer of one electron from the hydroquinone state of FMN to the [2Fe-2S] cluster (in black).
References
1. Dukor RK, Keiderling TA. (1991) Biopolymers 31(14):1747.
2. Arnesano F, et al. (2003) J Am Chem Soc 125(24):7200.
3. Shen Yet al. (2009) J Biomol NMR 44(4):213.
4. Bertini I, et al. (2001) J Biomol NMR 21(2):85.