Technological and social progress has been driven by the mastery of new materials. Think of the massive change in our way of life brought about by the invention of steel or the profound social changes stemming from the fabrication of controlled defects in silicon and the mastery of magnetic materials on the nanoscale, which together have brought about the information revolution. The age of big data is upon us, and for the third time in history magnetism is changing the world – first with the compass in the 11th century, then with the electrification of the planet in the 19th and now with the storage of more data each year than was ever recorded in all previous human history.
Magnetism in solids originates mainly from the intrinsic angular momentum borne by the electrons, known as their spin. According to quantum mechanics, this angular momentum measured in a particular direction can have only two values, equal in magnitude but opposite in sign; spin is either up or down. In a ferromagnetically-ordered material like iron, each atom has unequal numbers of spin-up and spin-down electrons – the difference in iron is about two - and the net atomic moments are stable in time and all are aligned. Early applications of ferromagnetic materials relied on the stray magnetic fields created outside the ferromagnet by the net spin polarization of the atoms. An abrupt change of focus came with the discovery of magnetic effects on electrical conduction, especially the giant magnetoresistance (GMR) and tunnel magnetoresistance (TMR) of magnetic thin film structures. In these devices, what counts is the degree of spin polarisation PF of the mobile electrons at the Fermi level, rather than the overall average. These devices have given birth to the new field of spin electronics.
Besides ferromagnets, where spins on neighbouring magnetic ions are all aligned parallel, there is another class known as antiferromagnets where they are aligned antiparallel, in a chequerboard pattern with no net magnetization. In classical antiferromagnets like NiO, the magnetic sublattices are chemically and structurally equivalent. A more useful variant is found in a class of materials known as ferrimagnets, where the sublattices of neighbouring atoms are structurally and/or chemically different, giving rise to a different magnetic moment for each sub-lattice therefore, like iron, a net magnetization. The common dark brown ferrite magnets that stick to the fridge are ferrimagnets. Ferrimagnets can be insulators, like ferrites, or metals with spin-polarized electrons at the Fermi level. This group of ferrimagnets is potentially very interesting because if the two sub-lattices exactly cancel their respective magnetic moments giving zero net moment, the material can remain spin polarized, retaining a finite spin polarisation at the Fermi level. Such a zero-moment ferrimagnet was predicted by de Groot in 1990, and a number of candidate materials have since been proposed by theoretical calculations.
For many years, all efforts to make a zero-moment ferromagnetic metal were unsuccessful. Either the material wouldn’t form in the crystallographic structure predicted, or the moments on the atoms on the individual sublattices completely disappeared. In January 2014 this changed. Researchers from Trinity College Dublin in collaboration with scientists from SOLEIL have found a material that seems to fit the bill. Thin films of the alloy Mn2Ru0.5Ga order in the L21 structure where magnetic Mn atoms occupy two non-equivalent crystallographic sites giving rise to a perfectly compensated zero-moment ferrimagnet. Seen from the outside, this material does not look like magnet – there is no stray field. But it is magnetically ordered, and the electrons at the Fermi level are highly spin polarized. The measured spin polarisation PF is in excess of 50%, a value higher than that of the common ferromagnetic metals Co, Fe and Ni. Furthermore, the sign of the magnetization can be changed by tuning the Ru concentration around 0.5.
This newly discovered material may prove to be very useful for spin electronics. We have a material where spin-polarized currents can flow which neither creates a stray field nor is it sensitive to any externally applied magnetic field. It is unnecessary to shield the material from external fields, and any information written in it cannot be accidentally erased. In memory applications where the storage density is of paramount importance, the lack of a stray field is advantageous as the state of one memory cell will not influence its neighbours. Nevertheless, it may be possible to store information in the zero-moment ferrimagnet by using spin polarized currents to record it. Although the value of spin polarisation PF ≈ 50% falls short of the 100% expected theoretically for a half-metal at absolute zero, there are prospects for improvements by working on the sample quality. The Mn2Ru0.5Ga, now known simply as MRG, is the first example of a whole new class of materials, which could help spin electronics to grow up.
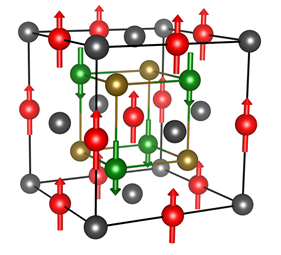
Figure 1. Model of an idealized Mn2RuxGa cell where x=1 and all Ru sites are occupied. Mn is present in two different sites (green and red), Ga and Ru are in grey and brown, respectively. The total atomic spins are drawn as arrows and they are localized on Mn sites.
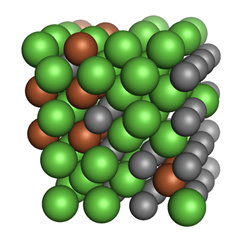
Figure 2. Super cell (116 atoms) of the periodic model used for Reverse Monte Carlo refinement of EXAFS data of Mn, Ru and Ga in Mn2RuxGa, x=0.5. Ga and Ru remains on separate atomic planes, while vacancies (only half of the Ru sites are occupied) and site occupations in layers are randomly distributed.
Grey spheres stand for Ga atoms, brown for Ru and green for Mn (independently of site occupied).
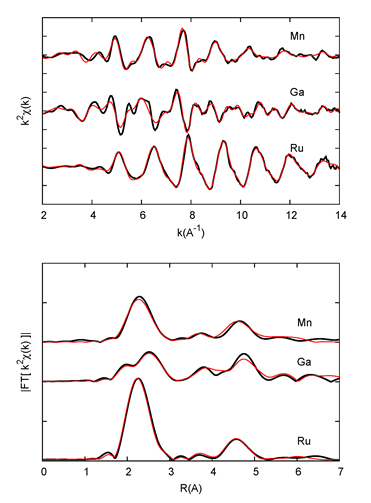
Figure 3. EXAFS data (black) and model (red) signals are drawn in the top panel, their respective Fourier transforms are drawn in the bottom panel. These data have been used to determine the atomic structure shown in Figure 2.