SOLEIL II - Come to SOLEIL
- Phonebook & CONTACTS
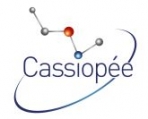
The CASSIOPEE beamline is dedicated to photoemission experiments in the 8 eV-1500 eV photon energy range. The beamline uses two undulators, and the main optical elements are the entrance optics and the monochromator. After the monochromator, the beamline is divided into two branches, providing photons to two endstations (Spin-resolved Photoemission, and High Resolution Angle-resolved Photoemission), both connected to a Molecular Beam Epitaxy chamber.
The CASSIOPEE beamline is dedicated to photoemission experiments in the 8 eV-1500 eV photon energy range. The beamline uses two undulators, and the main optical elements are the entrance optics and the monochromator. After the monochromator, the beamline is divided into two branches, supplying photons to two endstations (Spin-resolved Photoemission, and High Resolution Angle-resolved Photoemission), both connected to a Molecular Beam Epitaxy chamber.
Team
Aucun membre à afficher.
NICOLAOU Alessandro
PhD + Post-Doc
SEXTANT beamline - Synchrotron SOLEIL, France
SROUR Waked Daniel
PhD
OHTSUBO Yoshiyuki
Post-Doc
Osaka University, Japan
PALACIO-RODRIGUEZ Irene
Post-Doc
I.C.M.M. Madrid, Spain
PINON Julien
Technician
Synchrotron SOLEIL, France
NEGGACHE Amina
PhD
Technical data
Energy range
8 – 1500 eV
Energy resolution
E/ΔE from 20000 to 70000 as a function of photon energy
Source
- Electromagnetic undulator HU256 (8 - 155 eV)
- Apple II undulator HU60 (100 - 1500 eV)
Flux on first mirror
1.1015 Phot/s/0.1% bw
Optics
3-mirrors set entrance optics
Plane-grating Monochromator (3 Variable Line Spacing-Variable Groove Depth gratings, working in the modified Petersen mode)
Two endstations are present on the beamline:
- Spin-resolved Photoemission endstation: Toroidal miror focussing (250x200 to 720x350 µm2 beam spot)
- High-resolution Angle-resolved Photoemission: Pseudo-Wolter focussing (40x20 to 100x100 µm2 beam spot)
Flux on the sample
2.1013 Phot/s/0.1% bw
Polarisation
Variable Polarisation (Circular, Linear horizontal and vertical)
Beam spot size on the sample
- Spin-resolved Photoemission endstation: 250x200 to 720x350 μm2
- High-resolution Angle-resolved Photoemission endstation: 40x20 to 100x100 μm2
Sample Environment
3 ultra-high vacuum chambers:
- Molecular Beam Epitaxy chamber with surface science equipments (LEED, AES, RHEED, sputtering)
- Spin-resolved Photoemission endstation: Temperature from 25 to 400K
- High-resolution Angle-resolved Photoemission endstation: Temperature from 4 to 400K
Detectors
- Spin-resolved Photoemission endstation: Scienta SES2002 coupled to a Mott-detector
- High-resolution Angle-resolved Photoemission endstation: Scienta R4000 with wide-angle lenses
Scientific opportunities
Low dimension systems | Graphene, topological insulators... |
---|---|
Complex Oxides | Cobaltates, Pnictides, Cuprates, Vanadates, multiferroïcs... |
Surfaces and Interfaces |
Semiconductors Interfaces (Sn/Ge, Sn/Si, Alkali/Si:B), molecules/surfaces, biological species/surfaces... |
Spintronics | Magnetic tunnel junctions, Heusler compounds, Functional oxides interfaces... |
The CASSIOPEE beamline uses two undulators. The different optical elements are:
- The entrance optics
- The monochromator and its plane gratings
The beam can be directed at two different endstations:
- Spin-resolved PhotoEmission Spectroscopy (Spin-resolved PES)
- High-resolution Angle-resolved PhotoEmission Spectroscopy (HR-ARPES)
Both are connected to a Molecular Beam Epitaxy chamber (MBE)
Undulators
Two undulators are necessary to cover the large photon energy range available at Cassiopée. They were designed and characterized by the SOLEIL Insertion device Group (Antoine Daël, Oleg Chubar, Fabrice Marteau, Chamseddine Benabderrahmane, Marie-Emmanuelle Couprie and Fabien Briquez).
For low photon energies, Cassiopée uses a HU256 electromagnetic undulator, with a 256 mm period, which was designed at SOLEIL and built at the Budker Institute of Nuclear Physics in Novosibirsk (Russia). This undulator provides linearly (vertical or horizontal) or circularly (left or right) polarized photon in the 8 eV-155 eV range (see Figure 1).
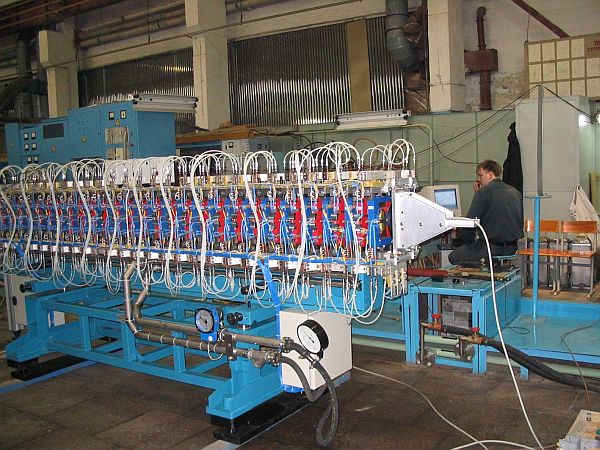
Figure 1: Photo of the low photon energy HU256 undulator.
For the high photon energies, Cassiopée uses a HU60 permanent magnet Apple II type undulator, with a 60 mm period, which was designed and built at SOLEIL. This undulator provides linearly (vertical or horizontal) or circularly (left or right) polarized photon in the 100 eV-1500 eV range (see Figure 2).
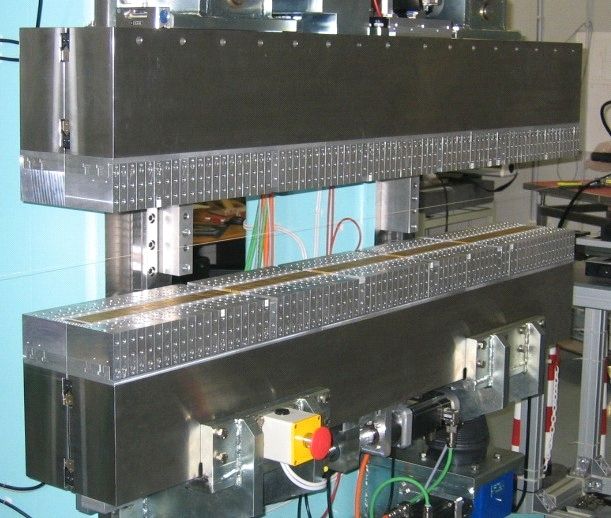
Figure 2: Photo of the high photon energy HU60 undulator.
Entrance Optics
The first optical elements play a key role in the beamline's stability and performance. Cassiopée entrance optics is made of three silicon mirrors (M1a is a plane mirror and M1b and M1c are two spherical mirrors) attached to the same rotating table whose rotation axis is vertical, in the plane of M1a surface. Ideally, the surfaces of the three mirrors should be parallel (see Figure 3).
These three mirrors (and especially M1a) have to absorb the power radiated by the ring and the insertion devices, without suffering any deformation. In the worst condition, this absorbed power can be as high as 250W. Therefore, these three mirrors are cooled down at a temperature of 100 K, where the silicon dilatation coefficient is zero, to avoid any thermal bump at the impact point of the photon beam. This is done by a 9 bars liquid nitrogen closed loop circulation.
The entrance optics has two working positions:
- A low energy position, in the 8 eV-155 eV photon energy range, when HU256 is used. In this case, the rotating table is set for an incident angle of 5.02° on M1a. The photon beam is then reflected towards M1b, whose radius is calculated to focus the HU256 source point onto the monochromator’s gratings.
- A high energy position, in the 100 eV-1500 eV photon energy range, when HU60 is used. The rotating table is then set for an incident angle of 2.44° on M1a. The photon beam is then reflected towards M1c, whose radius is calculated to focus the HU60 source point onto the monochromator’s gratings.
When going from one position to the other, an in-vacuum motor can also be used to rotate only M1a, in order to adjust the parallelism between M1a and the other concerned mirror. These two reflections shifted the useful VUV radiation axis from 62 mm in the horizontal plane. Hence, a tungsten rod can be placed along the undulators axis to block the gamma rays emitted by the ring (which go through the silicon mirror).
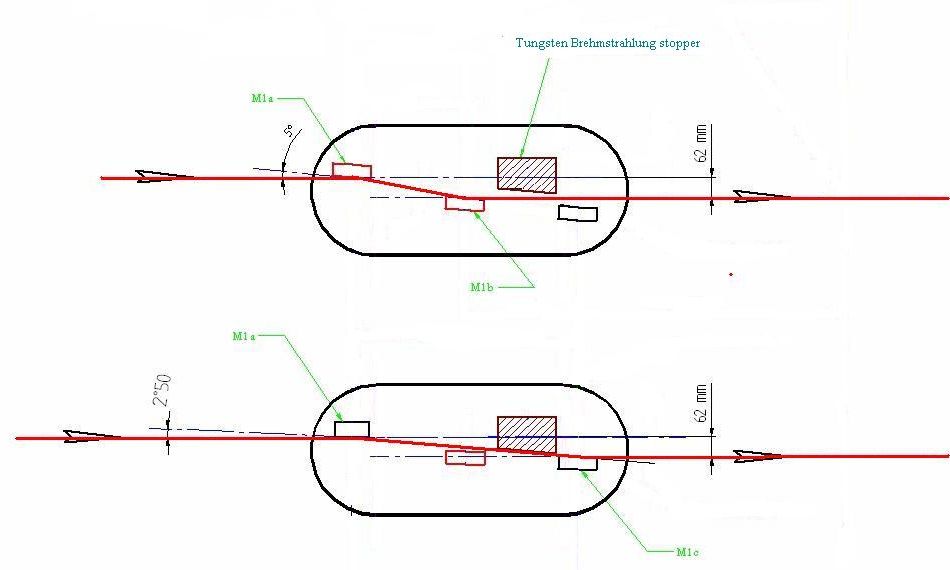
Figure 3: Principle of the Cassiopée entrance optics, with the low- and high-energy working positions. The entrance optics was built by the Jobin-Yvon company (Longjumeau, France).
Monochromator
Monochromator Geometry
The design of the CASSIOPEE plane grating monochromator was done by the SOLEIL Optics Group. The starting point is the well-known SX700 monochromator, but several modifications have been made.
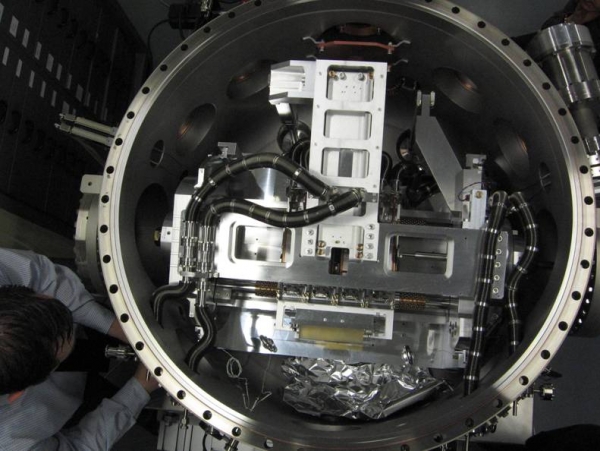
Figure 1: Picture showing the inside of the CASSIOPEE monochromator, with the plane mirror cage above the four available grating cages. The monochromator was built by the BesTec company (Berlin, Germany)
The working principle of the monochromator is shown in Figure 2. One can choose the incident angle α of the white beam on the plane grating by rotating the grating around an horizontal axis. The β angle can be chosen by rotating the plane mirror, above the grating, around a horizontal axis located below the grating plane, to ensure a constant height of the outgoing beam. For a given couple (α, β), the selected wavelength λ is given by the gratings law:
cos α - cos β = N.k.λ
where k is the diffraction order (-1 for CASSIOPEE) and N is the number of lines/mm on the grating. The CASSIOPEE monochromator contains three different gratings with 400 l/mm, 800 l/mm and 1600 l/mm.
The CASSIOPEE monochromator is designed to work in the “modified Petersen mode”, which means that α and β are always chosen to fulfil the following equation:
sin β ⁄ sin α = constant ≈ 0.2
to ensure an optimal resolution on the whole photon energy range.
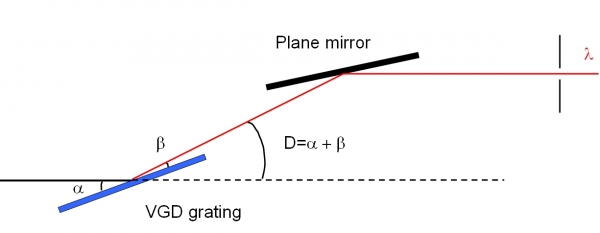
Figure 2: Working principle of the monochromator
Monochromator Gratings
The gratings of the CASSIOPEE beamline focus the photon beam by using varied line spacing (VLS). The groove depth also varies along the direction perpendicular to the beam. Hence, by choosing the position of the grating with respect to the beam, one can choose the groove depth seen by the beam to enhance the grating reflectivity at each photon energy. They were made by the Jobin-Yvon company (Lonjumeau, France).
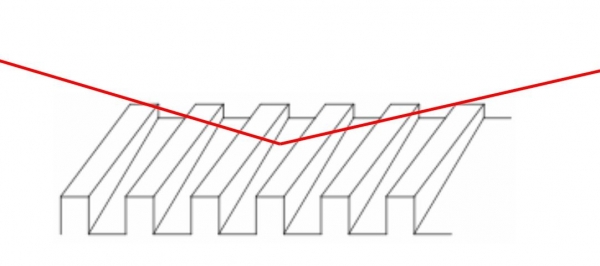
Figure 3: Schematic view of the variable groove depth grating.
Overview of the three Cassiopée experiments
The three Cassiopée experiments are:
- Spin-resolved Photoemission experiment
- High Resolution Angle-resolved Photoemission experiment
- Molecular Beam Epitaxy chamber
These chambers are connected to a Crossway chamber, and samples can be transferred in UHV from one chamber to the other as shown on Figure 1.
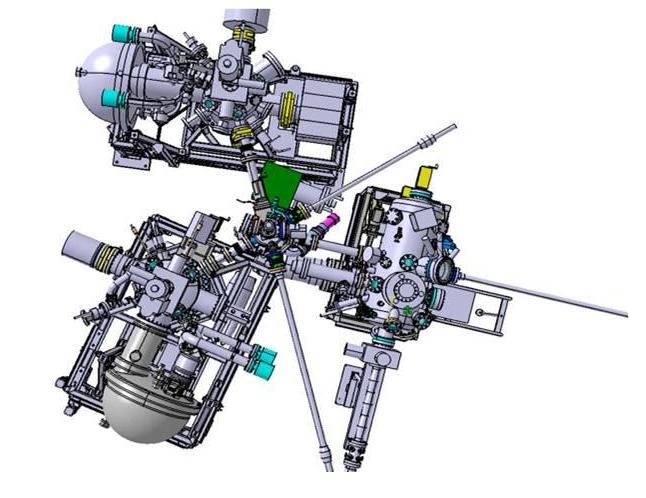
Figure 1: General view of the Cassiopée experiments, with the Spin-Resolved Photoemission experiment on top, the MBE chamber (bottom right) and the High Resolution Angle-resolved Photoemission experiment (bottom left). These three chambers are connected to the Crossway chamber, in the middle.
The samples are mounted on Omicron-type plates. See this section Users information for practical details.
Spin-resolved PhotoEmission Spectroscopy
Detectors
The Spin-Resolved Photoemission experiment is equipped with a Scienta SES2002 electron analyzer, which has been modified by the Scienta company to incorporate a Mott detector for spin analysis (see Figure 2). Part of the electron beam is sent towards the usual Scienta 2D detector, while the rest goes towards the Mott detector through transfer optics. The analyzer is mounted with horizontal slits. Angular resolution is then achieved in the horizontal direction. To accept the Mott detector, the 2D detector has been reduced to 1”, thus reducing the angular acceptance of the analyzer to ±8°.
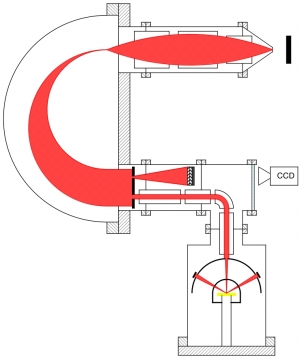
Figure 2: Schematic view of the Scienta SES 2002 and its transfer optics to the Mott detector.
Inside the Mott detector, the electron beam is scattered by a gold target, and the scattering asymmetry is measured parallel and perpendicular to the sample surface, thus measuring the magnetization components in these two directions. The Mott detector was built in the University of Edinburgh, by Prof. Murray Campbell (see Figure 3).
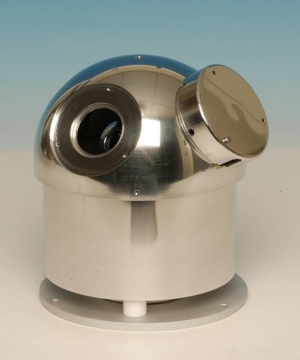
Figure 3: The Mott detector without one of the four channelplates.
Sample Environment
The Spin-Resolved Photoemission experiment is equipped with a 4-axis manipulator, with a motorized Z translation, manual X and Y translations, and manual θ rotation (see Figure 4). The sample can be cooled down to 40 K with a liquid He cryostat.
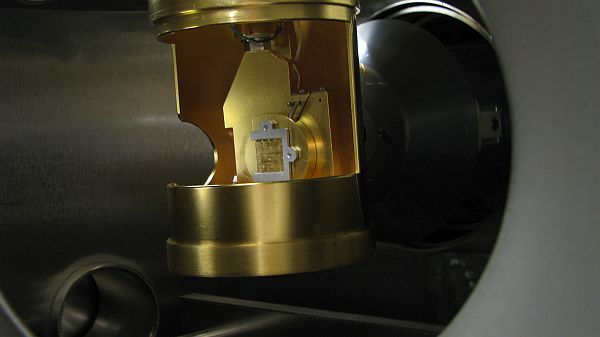
Figure 4: View of the Spin-Resolved endstation manipulator with the sample holder and the Scienta SES2002 electron analyzer.
A new in-situ magnetization system is underway.
Beam Size
The beam is sent to the Spin-Resolved Photoemission experiment by a toroidal mirror which also focuses the beam. The beam size is quite large, not to damage the sample during long acquisitions.
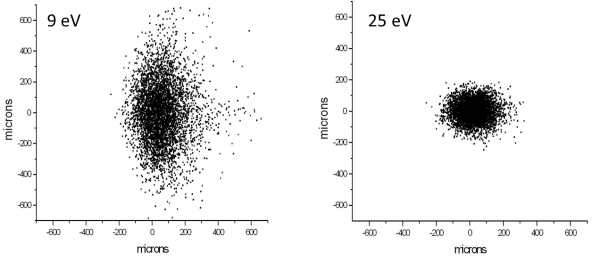
Figure 5: Theoretical beam sizes for two different photon energy.
High-resolution Angle-resolved PhotoEmission Spectroscopy (ARPES)
Detectors
The Cassiopée High Resolution Angle Resolved Photoemission experiment is equipped with a Scienta R4000 electron analyzer, whose angular acceptance is ±15° (Scienta Wide Angle Lens). This analyzer can be mounted with its slits either vertical or horizontal.
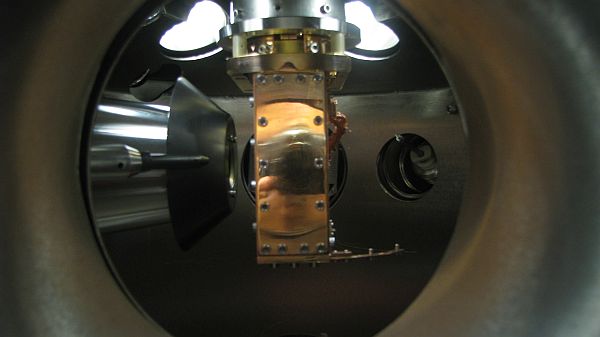
Figure 6: View of the ARPES endstation manipulator with the sample holder and the Scienta R4000 electron analyzer.
Sample Environment
The High Resolution Angle Resolved Photoemission experiment is equipped with a fully-motorized 4 axis sample holder with X, Y and Z translation and a θ rotation. The sample can be cooled down to 5 K with liquid He cryostat. The Scienta analyzer is mounted with vertical slits. The 30° vertical angular acceptance and the θ rotation of the sample allow to scan a large part of the reciprocal space (see Figure 6).
Beam Size
The beam is focussed by a pseudo-Wolter optics located just before the experimental chamber. It consists in a spherical mirror followed by a toroidal mirror (see Figure 7).
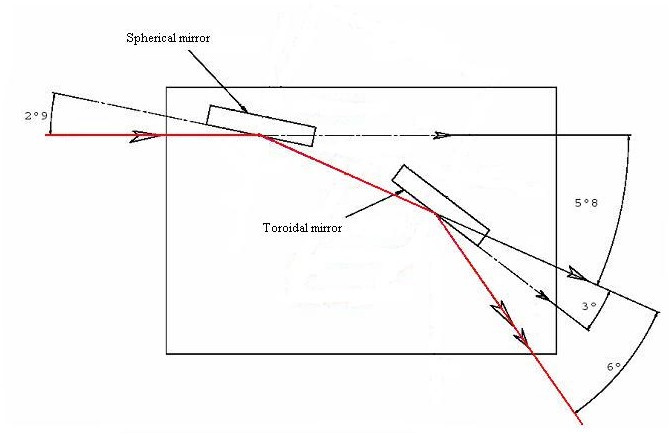
Figure 7: Schematic view of the pseudo-Wolter, focussing the beam in the CASSIOPEE High Resolution Angle Resolved Photoemission experiment.
The size of the beam depends on the photon energy, but is always of tens of microns. Ray tracing calculations are show in Figure 8.
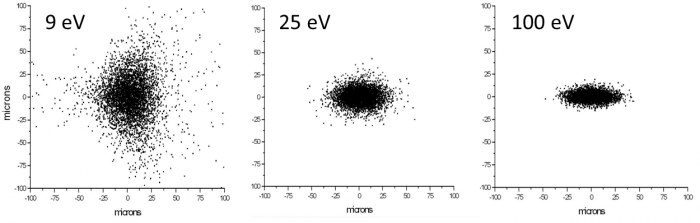
Figure 8: Theoretical beam sizes as a function of photon energy.
Molecular Beam Epitaxy Chamber (MBE)
The Cassiopée MBE chamber is equipped for surface preparation and characterization, as well as for thin film deposition (see Figure 9):
- 3-keV ion gun (OCI IPS3-D)
- Cylindrical mirror analyzer for Auger spectroscopy (Staib ESA100)
- LEED (Omicron SpectaLEED)
- RHEED (SPECS RHD30)
- 10 crucibles 3kW e-gun evaporator (Thermionics)
- Transferable electron bombardment evaporator (Omicron EFM3)
- Quartz microbalances (Inficon IC/5 controller)
Sample Holder
The MBE sample holder has three positions for the sample (see Figure 10):
- Position 1: Electron bombardment heating (up to 1000°C) and azimuthal rotation for RHEED experiment.
- Position 2: the sample can be cooled down to 80K by a liquid nitrogen circulation.
- Position 3: Direct current heating (for semi-conductors).
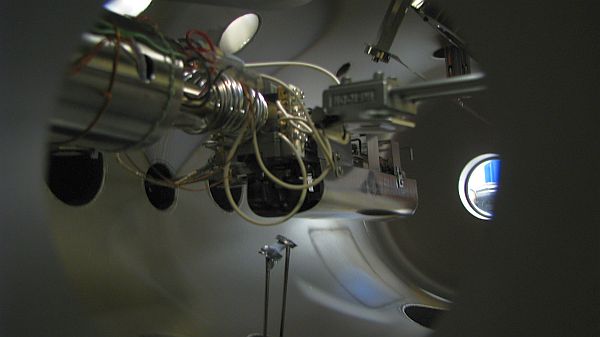
Figure 10: Inside view of the MBE chamber showing the sample holder and a quartz microbalance on the right.
Sample Holders
Samples are mounted on Omicron-type plates (see Figure 1a). They can be of different thicknesses to maintain the manipulator rotation axis in the plane of the sample surface (see Figure 1b), which is crucial for ARPES measurements. In any case, the total thickness (plate + sample) must be 3 mm.
Figure 1: Flat Omicron-type plate with tapped holes (a) and thickened plate (b). The maximum size of the sample is 12x12 mm² since 2 mm should be left uncovered on every side.
We also have special plates for direct current heating (see Figure 2) that can also be used in the in-situ bias application area (under construction).
Figure 2: Omicron-type plate for direct current heating. The maximum size of the sample is 7x7 mm² in this case.
Sample Loading and Storage
Loading Samples
The samples can be quickly introduced in UHV thanks to a load-lock mounted on the crossway chamber (see Figure 3).
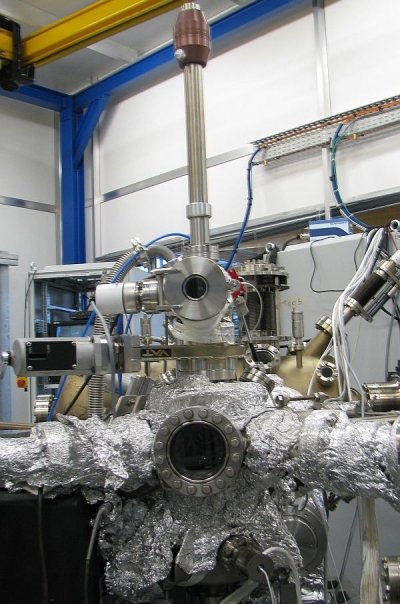
Figure 3: The crossway chamber with the load-lock on top.
Storing Samples
Inside the crossway, samples are stored on a carousel which can contain up to 16 samples (see Figure 4).
Figure 4: View of the carousel inside the crossway chamber.
Shipping Goods
Ligne CASSIOPEE
Synchrotron SOLEIL
Orme des Merisiers BP 48 - Saint Aubin
91192 - Gif-sur-Yvette, FRANCE
Downloads
